Is 3D Bioprinting the Future of Tailor-Made Medicine?
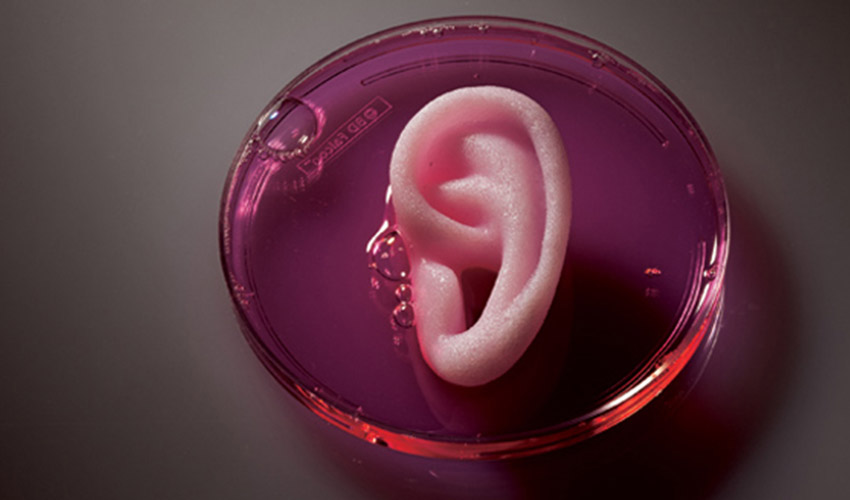
3D bioprinting is one of the most exciting applications that can be found for 3D printing in the healthcare sector. Until recently, the market was focused primarily on North America, however, many companies, laboratories and universities around the world are exploring this field too. Thanks to 3D printing-like techniques, cells and biomaterials can be combined and deposited layer by layer to create biomedical parts that have the same properties as natural tissues. During this process, various bioinks can be used to create tissue-like structures, which have applications in medical and tissue engineering fields. Of course, many know that the biggest endeavour of this field is to successfully bioprint a fully functional human organ.
While this technology is considered to be the future of medicine, there are still many unknowns attached to this printing process. Below, we will explore this topic and some of the recurring questions people have about bioprinting. In addition, we will also explore the different printing processes associated with this technology.
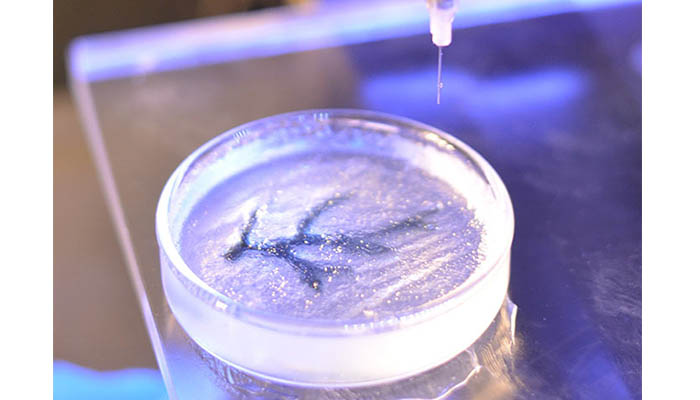
Image via FluidForm
It’s a known fact that demand for transplants continues to rise every year. In the United-States alone it’s 113,000 people that were on the transplant waiting list in 2019. Given that each year the number of people on the waiting list continues to be much larger than both the number of donors and transplants, the solution seems to be pointing to 3D bioprinting. As a matter of fact, an important breakthrough marked the medical field back in April. A team of researchers from Tel-Aviv University (TAU) successfully 3D printed a heart using human cells. This heart completely matched the immunological, cellular and anatomical properties of a human patient. Even though it consisted of the size of a rabbit’s heart, its complexity was a complete first: “People have managed to 3D-print the structure of a heart in the past, but not with cells or with blood vessels. Our results demonstrate the potential of our approach for engineering personalised tissue and organ replacement in the future,” explained Prof. Tal Dvir who led the research on this study.
As you will have understood 3D bioprinters can create complex cell structures through a layering process. The technology was developed by scientists in the hope of creating fully functional organs.
The Beginnings of 3D Bioprinting
3D bioprinting dates back to 1988, when Dr. Robert J. Klebe of the University of Texas presented his cytoscribing process. A method of micropositioning cells in order to construct two and three-dimensional synthetic fabrics using a common inkjet printer. In 2002, Professor Anthony Atala of Wake Forest University created the first organ using bioprinting: a small-scale kidney. To continue to foster more innovations in bioprinting, Organovo – the first commercial laboratory – arrived in 2010 in SanDiego, California. The lab quickly began working with Invetech developers to create one of the first bioprinters on the market, the NovoGen MMX.
Organovo has positioned itself as one of the leaders in the industry, they continue to work on developing bone tissue breakthroughs, such as when they created the hepatic transplantation tissue. Following the breakthrough from the team of Tel-Aviv University researchers, BIOLIFE4D was also able to bioprint a miniature human heart, making it the first company in the U.S. to achieve this. We expect more companies and research groups will be able to achieve this in the coming months.
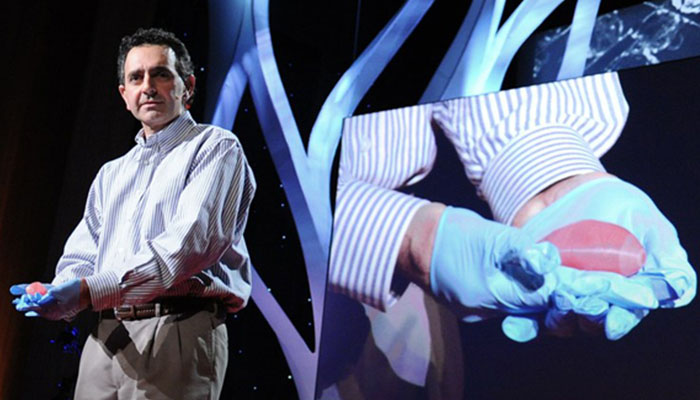
Anthony Atala
One of the biggest challenges is the high cost of development and lack of knowledge. However, new techniques are beginning to emerge to increase the chances of success and are divided into five different categories that we will be exploring below.
Inkjet Bioprinting
This technology is based on the classic inkjet printing process. Today, FDM 3D printers are being modified to achieve a similar printing process. This method makes it possible to deposit droplets of bio-ink (also known as biomaterials or biotins) layer-by-layer onto a hydrogel support or a culture plate. This technology can be classified into thermal and piezoelectric methods, both based on a form of biotin.
With thermal technology, it utilizes a heating system that creates air bubbles that collapse and provide the pressure needed to eject the “ink” drops. In contrast, the piezoelectric technology does not use heat to create the needed pressure. Instead, it uses an electric charge that accumulates in certain solid materials. In this case, a polycrystalline piezoelectric ceramic is present in each nozzle. A drawback with this last technology is that it can cause damage to the cell membrane if it is used too often.
Scientists have made great strides in regards to the patterns of molecules, cells and organs with inkjet printing. Molecules such as DNA have been successfully duplicated, making it easier to study cancers and potential treatments. Cells that help combat against breast cancer have also been successfully printed using inkjet bioprinting; Retaining their functions, with good prospects for creating living tissue structures or organs.
For Organovo, they rely on inkjet printing in order to create functional human tissues. Specifically, they are interested in reproducing the tissues found in the human liver. Their focus on this is in regards to the long waiting list for a liver transplant in the US. What Organovo hopes to do is to fix the damaged part of the liver, which would then provide a solution that would extend the life of the organ until the patient is eligible for a transplant. A waiting game that can sometimes take several years.
Extrusion Bioprinting
This method is based on the use of an extrusion to create 3D patterns and cellular constructions. Using biomaterials for printing, the solution is then extruded by coordinating the movement of a pressure-based piston or a microneedle over a stationary substrate. Printing consists of the printing of the model layer-by-layer, constructing itself together until the model is completed. The advantages that we find with this technology includes the ambient temperature process, direct cell incorporation and homogenous cell distribution. Some of the most popular bioprinters, such as the Bioplotter and the EnvisionTec, use this technique as it is considered to be the evolution from the inkjet bioprinting process.
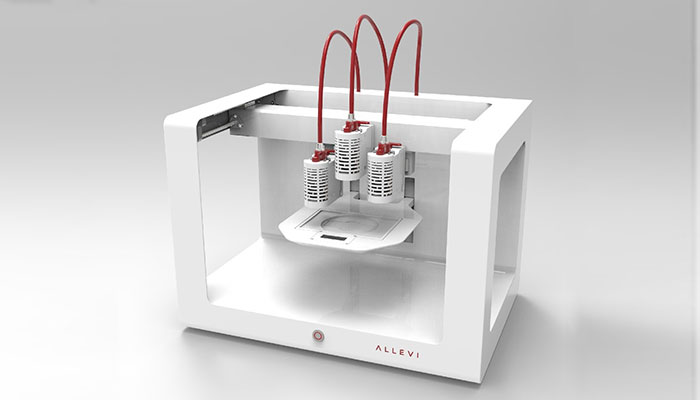
Allevi 3D bioprinter
Laser Assisted Bioprinting
This method uses laser as an energy source to deposit the biomaterials into a receptor. This technique consists of three parts: a laser source, a tape covered with biological materials and a receptor. The laser beams irradiate the tape, causing liquid biological materials to evaporate and reach the receptor in the form of droplets. These droplets contain a biopolymer, which retains the adhesion of the cells and helps the cell to begin growing. Compared to other technologies, laser-assisted bioprinting has unique advantages. Some specific advantages include it being a nozzle-free and contactless process that allows for high-resolution cell printing and droplet control Of biotin.
The French leader in bioprinting, Poietis, has launched a hair reproduction program in partnership with L’Oréal. The company uses the laser-assisted bioprinting technology, allowing them to deposit the cells precisely into a particular order. Today, the company is trying to recreate a hair follicle that could prove to be an effective solution to for stimulating hair grow, a potential alternative for men and women confronted with balding.
Stereolithography
STL technology consists of solidifying a photopolymer using ultraviolet light. It has the highest manufacturing accuracy and is suitable for bioprinting as it prints with hydrogels that are sensitive to light. This technology is still currently under development because there are still many limitations, such as lack of biocompatibility and biodegradability of polymers, adverse effects, and inability to the remove supports.
Bioprinting with Acoustic Waves
Developed by Carnegie Mellon University, Pennsylvania State University and MIT, this technology uses ‘acoustic clamps’, a microfluidic device that enables cells or particles to be manipulated using superficial acoustic waves. By using this device, researchers are able to manipulate where the waves will meet along each of the three axis. At these meeting points, the waves will then form a 3D trapping node that captures individual cells. These cells are collected in order to create 2D, and later 3D patterns. An overall technique that offers high performance in terms of movement accuracy.
Over time there have been more and more developments associated with this technology, with new applications or techniques debuting fairly quickly. An example of this can be seen at the Northwest University in Illinois with their 3D printed ovary as well as in Sweden, where researchers have successfully 3D printed human cartilage tissue. But while these advancements in the name of medicine have led to exciting talk and speculation about the future, there is another side that has yet to really be touched on, and that is the ethical implications we may face in regards to this technology.
The SWIFT Technique
Researchers from Harvard’s Wyss Institute for Biologically Inspired Engineering developed a new bioprinting technique called SWIFT (Sacrificial Writing Into Functional Tissue), as its name suggests this technique enables bioprinting blood vessels on living tissues. In other words, it 3D prints vascular channels into living matrices composed of stem-cell-derived organ building blocks (OBBs).
Rather than trying to 3D print an entire organ’s worth of cells, SWIFT focuses on only printing the vessels necessary to support a living tissue construct that contains large quantities of OBBs, which may ultimately be used therapeutically to repair and replace human organs with lab-grown versions containing patients’ own cells. In an experiment, organ-specific tissues that were printed with embedded vascular channels using SWIFT remained alive, while tissues grown without these channels experienced cell death within 12 hours.
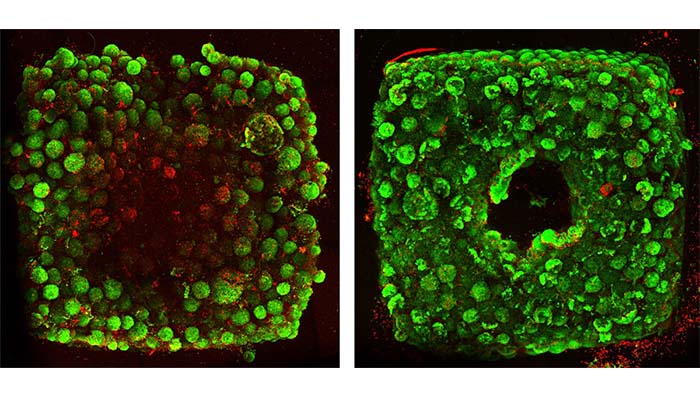
Organ-specific tissues that were printed with embedded vascular channels using SWIFT remained viable (right), while tissues grown without these channels experienced cell death within 12 hours (left).
The Future of Bioprinting
Biomedicine techniques seek to develop personalized medicine where doctors will be able to tailor treatments according to the needs of each patient. One of the main concerns of the industry is the costs associated with this personalization and who will be able to access it.
Another ethical difficulty is that it is impossible today to test the efficacy and safety of these treatments. After analyzing the different techniques used, we know that it is possible to develop functional organs that can replace human organs, but it is not yet possible to assess whether the patient’s body will accept the new tissue or the artificial organ. In addition to all of this, it is necessary to consider the legal regulations that must be put in place before these advances are made available to a wider audience.
Not to mention that new technologies can always be misused, and bioprinting is no exception. If technologies are capable of creating organs or tissues tailored to the specific needs of a human, considerations should be given as to the possible negative consequences of this tailored medicine. Specifically in reference to the potential for the creation of new superhuman capabilities, such as resilient bones or lungs that oxygenate differently. An attractive future for some people around the world, an even more attractive future for certain sectors, such as the military.
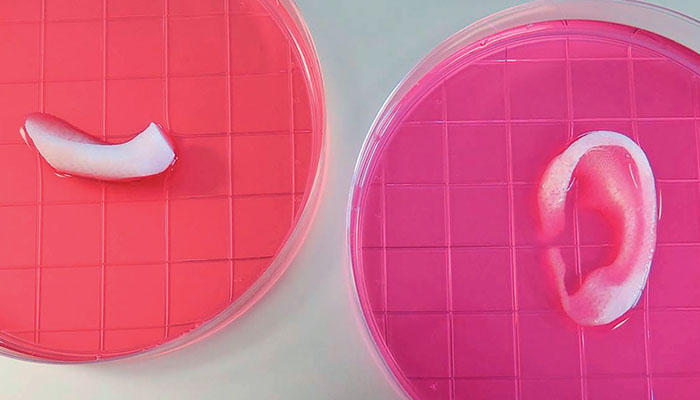
Image via CELLINK
Having discussed above the bioprinting techniques that we’ve observed on the market, it seems that even though the technology has a long way to go, printing body parts will be the next step in organ transplantation. In terms of the body parts we’ve seen be bioprinted we can name bones, corneas, cartilage, hearts and skin. These research projects have taken place in various universities across the world, in the U.S., Europe and Asia.
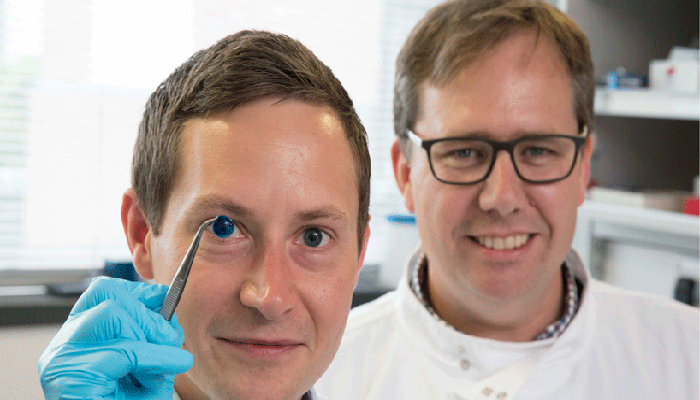
The two creators of the 3D printed cornea | Image via Newcastle University
Grand View Research, an important market research firm based in San Francisco, revealed that it expects the global bioprinting market to reach $4.1 billion by 2026, registering a CAGR of 19.5%. The main players are expected to continue growing in North America, with the United States holding strong in the first position, followed by Canada. Many European countries are also bringing developments to bioprinting, with the current leader being the United Kingdom.
Today, it’s also the material segment that is expected to grow, thanks to other technologies such as AI, scientists can more easily determine the right biomaterial combinations for turning scaffolds into tissues. Bioprinting companies are expected to focus on developing more biomaterials, as well as bioprinting systems with more printheads to support using more bioinks on the same print. It’s also expected for bioprinting software to be refreshed, offering more capabilities for the user. Finally, it’s expected that more business sectors will get started with leveraging bioprinting capabilities in their market.
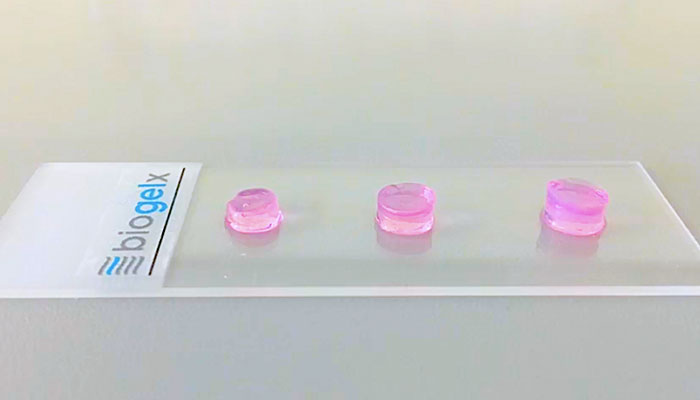
For example, the company Biogelx is developing tunable synthetic materials for 3D cell culture and 3D bioprinting applications | Image via Biogelx
While the main growth that will be seen in this market will focus on the development of tissues and organs, it is safe to say that in maybe a decade’s time, we will be talking more about 3D printed human organs and transplants. There are still many great things for researchers and scientists to discover in the future, but we are sure that 3D bioprinting may be one of the greatest medical developments that we will see in our lifetimes; a true revolution for the future of medicine.
Do you think that bioprinting is the future of tailor-made medicine? Let us know in a comment below or in a comment on our Facebook and Twitter page!